If you’re somewhat new to CFD, you might be asking yourself why there are so many turbulence models.
The answer lies at the heart of what CFD software does. It solves the Navier-Stokes Equation as well as the energy and mass conservation equations. When solving these equations, Prandtl realized that no direct mathematical solution existed for turbulent flow. This has been coined as the turbulence closure problem because the equations can’t be closed for turbulent flow.
As usual, the engineer’s solution to solving equations that can’t be solved involves simplification methods and observations. As always, these methods vary depending on the type of problem you’re solving, and what is considered important or conservative.
So how do you know which model to choose?
Choosing the correct model is important, and can have a big impact on the results. In this post, we’ll take a look at some of the most popular models, and their benefits and detractors.
RANS – Reynolds Averaged Navier Stokes Models
RANS based models are by far the most common type of turbulence modeling. These models use viscosity to close the Navier-Stokes equations. RANS models work by assuming that the turbulence is effectively isotropic, and not stretched by the proximity to the wall, or in strong swirling or shear flow. There’s a number of variants of RANS models, as we’ll discuss below.
RANS Single Equation Model: Spalart-Allmaras (SA)

The Spalart-Allmaras models are mainly used for aerospace and turbomachinery applications. It works well for supersonic and transonic flows over airfoils, boundary layer flows, etc.
Positives:
- Not Memory intensive
- Fast implementation
- Good convergence
- Forgiving of poor quality meshes – especially near walls
- Stanford notes that this is a good compromise for accuracy vs. simplicity
Negatives
- No wall functions
- Not good for shear flows
- Under-predicts separation (don’t use this for calculating stall)
- Can’t predict the decay of homogenous, isotropic turbulence
RANS Two Equation Model: k-ε

This is one of the most common types of turbulence models. They’re reasonably accurate for a wide range of applications.
Positives
- good for external flows
- Reasonable Accuracy
Negatives
- ε can’t be calculated near the wall, so wall functions are needed
- Poor performance for flows with strong separation, strong streamline curvature, and large pressure gradients
RANS Two Equation Model: Realizable k-ε

This is the workhorse of the turbulence models. It’s the most proven, widely documented, and most available turbulence model around. In fact, many of the commercial software packages have this model set to the default.
Positives:
- Planar surfaces
- Round jets
- Rotation
- Recirculation
- Streamline curvature
- Improved BL under adverse pressure gradients or separation
Negatives:
- More computationally Expensive
RANS Two Equation Model: RNG k-ε

The RNG k-ε model is an improvement on the standard k-ε model for shear flows, swirling flows and separation. It also works well for flows with high strain rates.
Positives:
- Swirling flow
- Shear flow
- Separation
- Low Re
- Underestimates value of k, creating less viscous and more realistic flow features with complex geometry
Negatives:
- This model has been superseded by others, even causing some vendors to stop using it in their software
RANS Two Equation Model: Standard k-ω
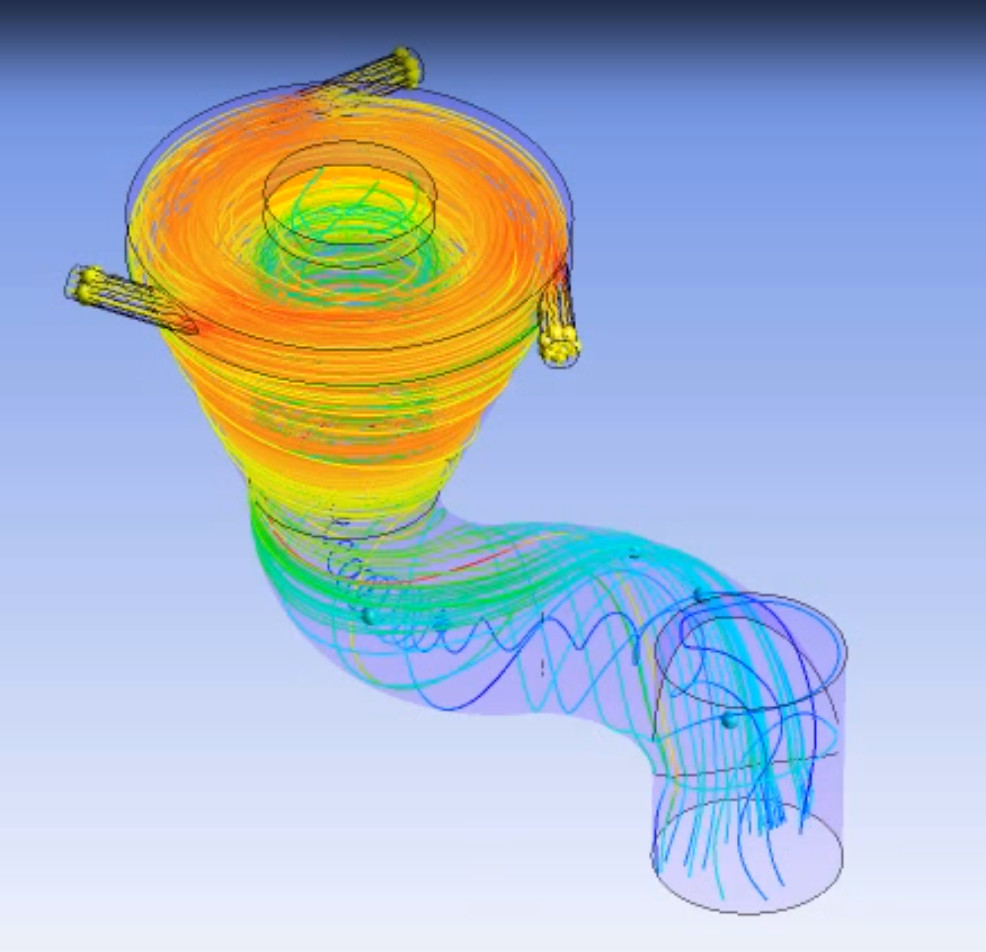
This model incorporates the rate of dissipation of the kinetic energy (ω). The goal here is to model the near-wall interactions better than the k-ε models.
Positives:
- Good for internal flows
- Turbomachinery/Aerospace
- Strong Vortices
- No wall functions are necessary
Negatives:
- RANS two equation model: Standard k-ω and SST k-ω
- Good for Internal flows
- Over-predict shear stresses of adverse pressure gradient BLs
- Over-Predicts Separation
- Issues with free-stream flows
- Difficulty converging compared to k-ε
- Sensitive to initial conditions
RANS Two Equation Model: Shear Stress Transport k-ω

The SST k-ω model is a hybrid of the best of k-ω and k-ε. This model uses the k-ω model near walls, and transitions to k-ε model in the open flow field. It’s popular in aerospace and turbo-machinery applications.
Positives:
- Separation and reattachment modeled better than k-ε and k-ω
- Enhancement to k-ω
- Can be applied to the viscous affected region without further modification
- Good to use when flow is too complex for Spartan-Allmaras
Negatives:
- Blending is arbitrary, and can mess up some critical features of turbulence
- Requires limiters to improve prediction of stagnant regions of the flow
- Does not take buoyancy into account
Large-Eddy Simulation (LES)

LES is much more complex than RANS models, and is better suited for high-end applications (combustion, mixing, external aerodynamics like flow around bluff bodies).
Large-eddy simulation (LES) models resolve the largest scales of turbulence and model the rest by use of sub-grid turbulence models or by blending with a RANS model.
Positives:
- Buoyancy
- Thermal fatigue
- Combustion
- Mixing
- Vibration
Negatives:
- Cannot predict turbulence near the wall (unless using a hybrid).
- Computationally expensive
Reynolds Stress Model

The Reynolds Stress Model is the most complete turbulence model with regards to representing turbulent flow. This is typically reserved for the most complex flows where the CFD analyst doesn’t know exactly what to expect (unstudied flows), or for extremely complex flows.
Positives:
- Captures complex strains like swirling flows and secondary flows
- Only accurate closure for swirling flows
Negatives:
- Computationally expensive
- Sensitive to initial conditions
- A lot of modeling required
- Requires high fidelity mesh
Engineering Judgement
The choice for turbulence model comes down to engineering judgement. If you’re not sure which turbulence model is accurate, consider running the simulation a few times with different models to see if one model is missing or inaccurately modeling some flow phenomena. Based on the information here, you should be able to at least narrow down the list of CFD models you should use for your analysis.