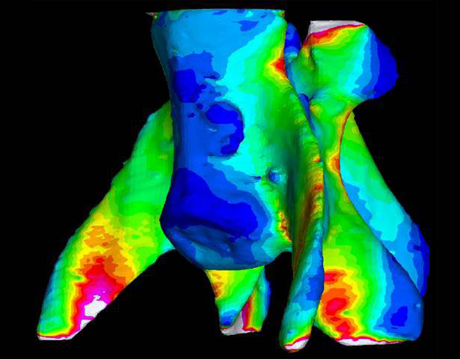
Pound on a bone with enough force and it’ll break. Just where, though, depends on whether or not bone behaves like other structural materials.
Researchers have long guessed it did—but they didn’t really know.
Bone is famously strong stuff, surpassing reinforced concrete when it comes to withstanding compression forces. (Exactly why we’re not laying foundations with skeletal remains, is perhaps, the subject of another article.)
How the spongy, matrix-like trabeculae on the inside works with the bone as a whole to produce this superhuman (yet human) strength remains a mystery. To solve it, researchers look to the breaking points.
Micro-CT scans offer us a deep, 3-D view of the porous, intricate trabecuale at the micro level. And they are bringing us closer to understanding how bone strength works at every scale. The digital output of the scans is easily converted into finite element models, which can be squished and twisted to test how the material stands up to various stresses.
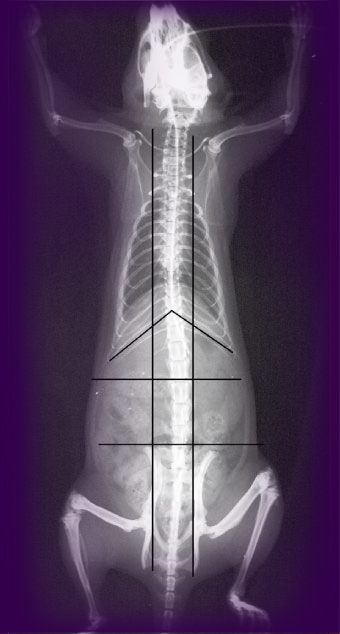
“Anybody, basically, can make an FE model and make it look good, with pretty pictures and all the colors,” says Naomi Tsafnat, a research associate at the University of New South Wales, “The question is: Is it accurate?”
Tsafnat decided to test that accuracy by running a compression test on the very rat vertebra that she planned to model.
The chief untested assumption that most previous FE modelers of bone have made (and, to be fair, disputed among themselves) is that at the microscale, the material is isotropic and homogenous.
When subjected to stress, it should, therefore, fail in the same way as a material like metal. “Not a bad assumption,” Tsafnat notes. But there were no experimental data to show that the assumption was anything other than just that.
Many programs that had been used to model bone incorporated this assumption (in the form of von Mises stress) to predict where it might fracture when subjected to various forces. But the formulation had only been proven accurate with materials like metal—there had as yet been no experiment to show that it made sense to do so with bone. In theory, the pretty pictures of stresses radiating through bones might have been wildly off.
So Tsafnat placed her rat bone between two clamps in a compression chamber. The apparatus was small enough to fit in the University of Sydney’s newly purchased Micro-CT scanner, enabling her to scan the bone before and after it was put through its paces.
“We had the exact geometry, and all the boundary conditions were set up. Our only unknown was the material properties themselves,” says Tsafnat. The model bone was then squashed to reveal breaking points. Then the real bone was squashed until it really broke.
Thankfully, the experiment had a happy ending (though not, perhaps, for the rat bone . . . or the rat). Tsafnat’s model was dead on, validating the assumptions made for many a scanned bone of yesteryear. The fracture points it predicted were indeed the very places where the real bone fractured.
“Now you can do ‘What if?’ simulations, and you can have confidence in your model, that it’s probably accurate,” says Tsafnat. “If you can validate it experimentally, that’s the best model you can get.”